Once having proved to the world that cloud seeding works, Israel no longer seeds to add water to the Sea of Galilee, its primary source of fresh water. This is a scientific story that has taken almost 60 years to play out.
Arthur L. Rangno, retiree, Research Scientist IV, Cloud and Aerosol Research Group, University of Washington, Seattle. Recipient in 2005 with Prof. Peter V. Hobbs of a small monetary award adjudicated by the World Meteorological Organization for studies in weather modification/cloud seeding.
ABSTRACT
The Israeli seeding program is traced from its modern inception in 1961 to the pinnacle of success it achieved after the statistically-significant results of the first two randomized experiments, Israel-1 and Israel-2, had been reported and how the early “ripe for-seeding” cloud reports laid the foundation for the virtually unanimous view that those experiments had provided proof that cloud seeding had significantly increased rainfall.
The peak was followed by an erosion of status that began with a cloud reports at variance with those of the experimenters in 1988 followed by the full reporting of Israel-2 crossover result in 1990 which revealed a null result. It had not repeated the apparent crossover success of the Israel-1 experiment. Moreover, reports for a third, 20 year long randomized experiment, Israel-3, in the early1990s suggested rain had been decreased due to seeding by 9%. This fall in status was accelerated when independent re-analyses of Israel-1 and 2 appeared in 1995 and a second independent reanalysis for the north target of Israel-2 in 2010 all of which found strong evidence of null results in these experiments.
Two independent evaluations of the operational seeding program that began for Lake Kinneret (Sea of Galilee) in 1975, found no indications that runoff had been increased by seeding. The program was terminated as a new randomized experiment, Israel-4 began.
The null results reported in these experiments and the operational seeding are supported by cloud studies over the past 30 years indicating that the clouds of Israel are generally unsuitable for seeding due to their high precipitating efficiency and the high temperatures (>-10°C) at which ice onsets in them.
A final seven season randomized experiment, Israel-4, conducted in the mountainous regions of the Golan Heights, ended in 2020 with a null result; no viable increase in rainfall had occurred on seeded days.
————————————————————————————
- The impact of the first two Israeli randomized cloud seeding experiments: cloud seeding proved.
“Almost every review of the status of weather modification published since 1970 has described the Israeli experiments as providing the most convincing evidence available anywhere that cloud seeding can, in fact, increase average rainfall over an area. The credibility of the reported rainfall increases from Israel I and Israel II is due to impressive compilations of statistics and to Dr. Gagin ‘s cloud physics studies, which provided a plausible explanationfor the rainfall increases suggested by the statistical analyses.” ————–A. S. Dennis (1989), part of his preface to the Memorial Issue of the J. Appl. Meteor.
No experiments in the history of cloud seeding have had more impact on the world of cloud seeding than the first two long-term randomized experiments conducted in Israel between 1961 and 1975 by scientists at the Hebrew University of Jerusalem (HUJ). The apparently successful results of these experiments, Israel-1 and Israel-2[1], were cited in numerous meteorology textbooks as singular successes in cloud seeding from introductory ones (e.g., Neiburger et al. 1982; Moran et al. 1991; Lutgens and Tarbuck 1995) to those at graduate levels (e.g., Wallace and Hobbs 1977; Dennis 1980; Young 1993). Moreover, it led to attempts at transferring the Israeli results to Spain (Vali 1988) and Italy (List et al. 1999) and influenced nearby Arab countries to try cloud seeding.
These two experiments were also hailed in reviews by expert scientific panels, organizations and by leading individual scientists in the field as cloud seeding successes (e.g., Sax et al. 1975; Tukey et al. 1978a; b; Simpson 1979; Grant and Cotton 1979; List 1980; Mason 1980; 1982; Amer. Meteor Soc. 1984; Silverman 1986; Cotton (1986); World Meteorological Organization 1986; 1988; 1992; Dennis 1989, Cotton and Pielke 1995). Typical of media reports was a summary of cloud seeding by Kerr (1982), who described the first two Israeli experiments in this way: “Cloud Seeding: One Success in 35 years[2].”
But should they have been that “one success”? Could all the glowing statistical results from the first two experiments, supported by seemingly solid cloud microstructure studies, in fact, be “scientific mirages”, as Foster and Huber (1997) termed faulty literature? And, as faulty literature, could it still be published in our peer-reviewed journals and be accepted as valid by our best scientists, national and international panels?
The answer to this question is, “yes.”
The story told here is one of the most compelling chapters in the field of cloud seeding, one that has taken almost 60 years to play out. And, as many findings are within the domain of cloud seeding (e.g., Changnon and Lambright 1990), it has been steeped in controversy until recently when the “dust” seems to have settled due to the recent null outcome of a fourth long term randomized experiment. In this case, the controversy has been between those from the institution from which the reports of seeding successes and cloud descriptions originated and the external skeptics who investigated those claims and found them lacking.
However, as of Freud et al. 2015, there is general agreement that the clouds of Israel exhibit a high precipitation efficiency with the onset of precipitation in clouds with tops between -3°C and -5°C, making them generally unsuitable for glaciogenic seeding. A hoped-for exception to this was in the mountainous Golan Heights of Israel where “Israel-4″ was carried out with disappointing results after seven seasons of randomized seeding.
I am well-acquainted with these experiments. As a skeptic of the experimenters’ many cloud descriptions, I traveled to Israel for an 11-week cloud investigation[3], from January through mid-March 1986. The results were published in Rangno 1988 (hereafter, R88). I also carried out re-analyses of the Israel-1 and 2 experiments (Rangno and Hobbs 1995a, hereafter, RH95a), the latter subject to numerous “Comments[4]” (Rosenfeld 1997; Dennis and Orville 1997; Woodley 1997; Ben-Zvi 1997 and “Replies” (Rangno and Hobbs 1997a, b, c, d, e).
This review begins with descriptions of the first two experiments, their initial convincing results followed by the associated “ripe-for-seeding” cloud descriptions. Overall, this review supports the views of Bruintjes (1999) and Silverman’s (2001) that the confidence that the Israel-1 and Israel-2 experiments had proven cloud seeding has waned due to later published work. Most of the material here was not included in Bruintjes (1999) or Silverman’s (2001) reviews these experiments which included cursory reviews of several other experiments[5].
- The rain season climate of Israel
The rain season in Israel runs from about mid-October through April and consists of about 50-70 days, the greater number in the north (Goldreich 2003). Showers form in cumuliform clouds as cold polar air masses exiting the European Continent move onto the warm waters of the Mediterranean Sea, enhanced into clusters or bands by traveling upper air troughs in the westerlies but are more scattered in coverage behind troughs as subsidence occurs. These events produce about 120 hours of measurable rainfall at each site (Goldreich 2003).
The air masses that move onto the Mediterranean from Europe contain considerable aerosol content as the pass over the Mediterranean Sea. However, as the clouds gain in stature much like lake-effect Cumulus clouds do, the increased mixing depth downstream helps reduce the impact of European aerosols; the initial high aerosol concentrations are dispersed over greater depths. In addition, these clouds also take up marine aerosols (Levin et al. 1996, Freud et al. 2015).
- Descriptions of Israel-1 and 2 randomized cloud seeding experiments.
a) About Israel-1
This first of three two daily[6]randomized cloud seeding experiments, begun in the late winter of 1961 had two targets, one of which was designated in advance to be seeded each day during the rain season of mid-October through April. The Israel-1 experiment of six and a half rain seasons was a “crossover” experiment in which the results of seeding are combined from the two target areas (e.g., Neumann et al. 1967; Gabriel 1967a; b). In a crossover experiment, one of the two targets is seeded every day; experimental data builds twice as fast compared to single target experiments (e.g., Neumann and Shimbursky 1972; Gabriel 1999).
The two seeding targets in Israel-1 were called, “north” and “center”, and were separated by a small “buffer zone” (BZ) that was left unseeded (Figure 1).
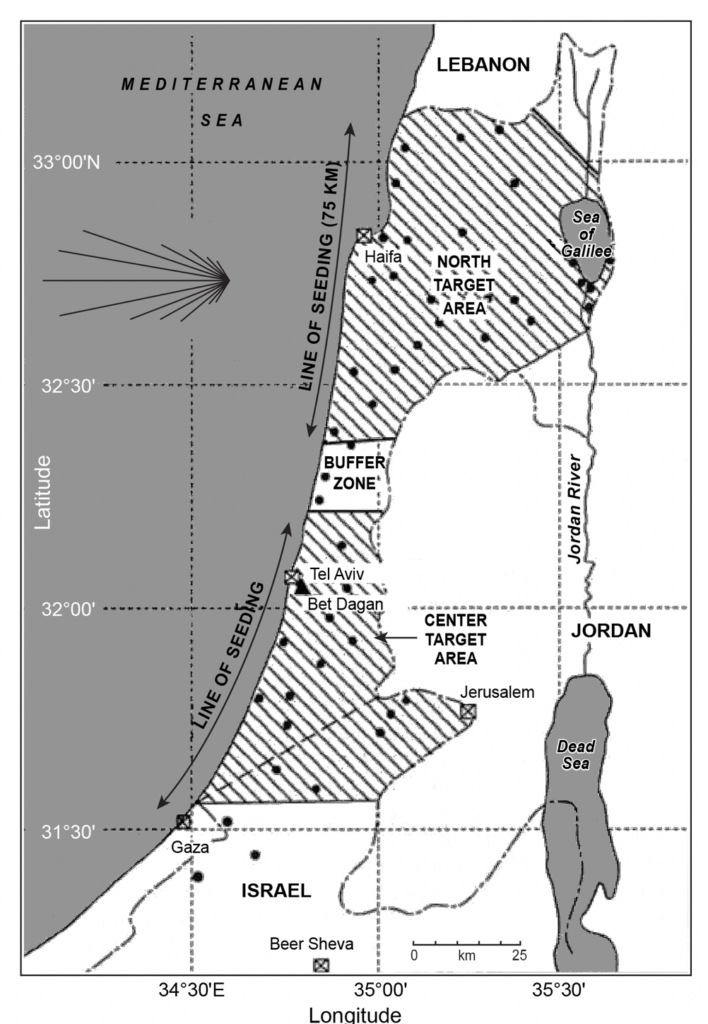
Figure 1. Map of Israel showing the north and center target areas (shaded) and the buffer zone for Israel-1. (after Gabriel 1967a). Thewind rose shows the percentage of the time that the 850-hPa wind was from a particular direction when rain was falling at the time of or within 90 min of, the rawinsonde launch time and at, or within 60 km of, the rawinsonde launch site.
Due to the proximity of the targets, significant correlation (~0.8) in rainfall existed between each one as established in historical comparisons (e.g., Gabriel 1967a). It is also assumed in crossover experiments that the natural cloud microstructure in the two targets is virtually identical and will respond to seeding in the same way.
The seeding in Israel-1 was carried out by a single DC-3 aircraft flying at about 50 m s-1parallel to and within about 10 km of the coastline releasing silver iodide (AgI) at or just below cloud bases. Cloud bases average about 700-800 m above sea level (Gabriel 1967a, Gagin and Neumann 1974, hereafter GN74a).
The line-seeding legs conducted upwind of each target at cloud base were 65-75 km each way. This required about 20-25 min to complete one roundtrip seeding cycle[7]. AgI released by the aircraft was expected to be ingested in updrafts to form the ice crystals needed to initiate precipitation by the Wegener-Bergeron-Findeisen (WBF) mechanism. In the WBF mechanism, ice particles grow in clouds containing supercooled liquid water or they grow where ice supersaturation exists even without the liquid phase. It was generally believed in the 1960s that within the cloud top temperature range of -10° C to -20° C, that there would be few if any ice crystals along with abundant supercooled liquid water in such clouds (e. g., Fletcher 1962; Mason 1971). The lower part of this temperature range is where AgI that was used in both Israeli experiments was highly active in forming ice crystals. Cloud tops during storms in the temperature range above were reported to be common from radar data accumulated in Israel-2 (e.g, Gagin and Neumann 1976, hereafter GN76).
Aircraft line-seeding was carried out for an average of 4 h per rain day for a total of about 70 h upwind of each target per entire rain season in Israel-1 (Gabriel 1967a, Table I). Of these hours, an average of 42 h was carried out in the daytime and 28 h at night (Gabriel and Neumann 1978)[8].
Seeding was conducted when a “cloud seeding officer” (Gabriel 1967a) determined that cloud tops were colder than -5°C, generally above 3 km MSL in Israel. At night, it was assumed cloud tops were colder than -5°C if rain was observed.
Israel-1 ended with the 1966-67 rain season with 378[9]experimental days (e.g., Wurtele 1971). The days culled were those that had measurable rain in the BZ in an effort to minimize the number of completely dry days in the targets[10].
b) Results of Israel-1
Interim results from Israel-1 were first reported by Gabriel (1967a, b; Neumann et al. 1967), followed by a report of the full experiment in a non-peer-reviewed, Final Report by Gabriel and Baras (1970). The complete Israel-1 experiment description first appeared in the peer-reviewed literature by Wurtele (1971). These reports indicated that a 15% increase in rainfall had been produced by seeding when the rainfall data from both the “north” and “center” targets were combined as the design specified. Larger rainfall increases on seeded days were noted in the center target compared to the north target (e.g., Gabriel 1967a), and the suggested increases in rain due to seeding were larger farther inland from the aircraft seeding line (e.g., Gabriel and Baras 1970; Wurtele 1971, Gagin and Neumann 1974a, and Gagin and Neumann 1974b, hereafter, GN74a and GN74b). This latter finding was compatible with seeding logistics and the time of formation of rain once the AgI reached the low cloud temperatures (<-10° C) required for appreciable activation, that level generally above 4 km ASL.
However, a discrepancy arose in the analysis by Wurtele (1971) that had not been noted in the analyses prior to 1970: the greatest of all the apparent increases in rainfall due to seeding was in BZ between the two targets on center seeded days. This discovery was later inferred by the experimenters as an unintended effect of seeding of the BZ on center seeded days (e.g., Gabriel and Baras 1970; GN74a). Wurtele (1971), however, had quoted the Chief Meteorologist of Israel-1, who stated that seeding could only have affected the BZ, “5-10% of the time” and “most likely less than 5%” of the hours that the center had been seeded.
In the meantime, Brier et al. 1974, in an independent re-analysis, expanded the apparent increases in rainfall due to seeding into Lebanon and Jordan, while Sharon (1978) in a study comparing the size of rain systems on seeded days in Israel-1, concluded that they were 10 km larger in area than on non-seeded days.
Except for the discrepancy in the BZ, Israel-1 was a very convincing outcome for a well-designed experiment. However, at this time, little was known about the clouds of Israel. This was to change during Israel-2 when descriptions of Israeli clouds began to appear in the literature, ones highly supportive of potential for seeding (e.g., Patrich and Gagin, 1970; Gagin 1971, Gagin and Steinhorn 1974, Gagin and Neumann 1974).
c) About Israel 2
Israel-2 was carried out from 1969-70 through 1974-75 rain seasons. It was also designed as a crossover experiment patterned after Israel-1 in which random seeding took place in two target areas, this time called “north” and “south” (e.g., GN74a, Silverman 2001). The north target was shifted inland from Israel-1, as was the aircraft line seeding path to improve targeting of the Sea of Galilee (a.k.a., Lake Kinneret) watershed, Israel’s primary natural fresh water source. The south target area included the area of the “center” target of Israel-1 as well as a large area to the south of the former “center” target (e.g., GN74a). The same BZ as in Israel-1 was used in Israel-2 (Figure 2).
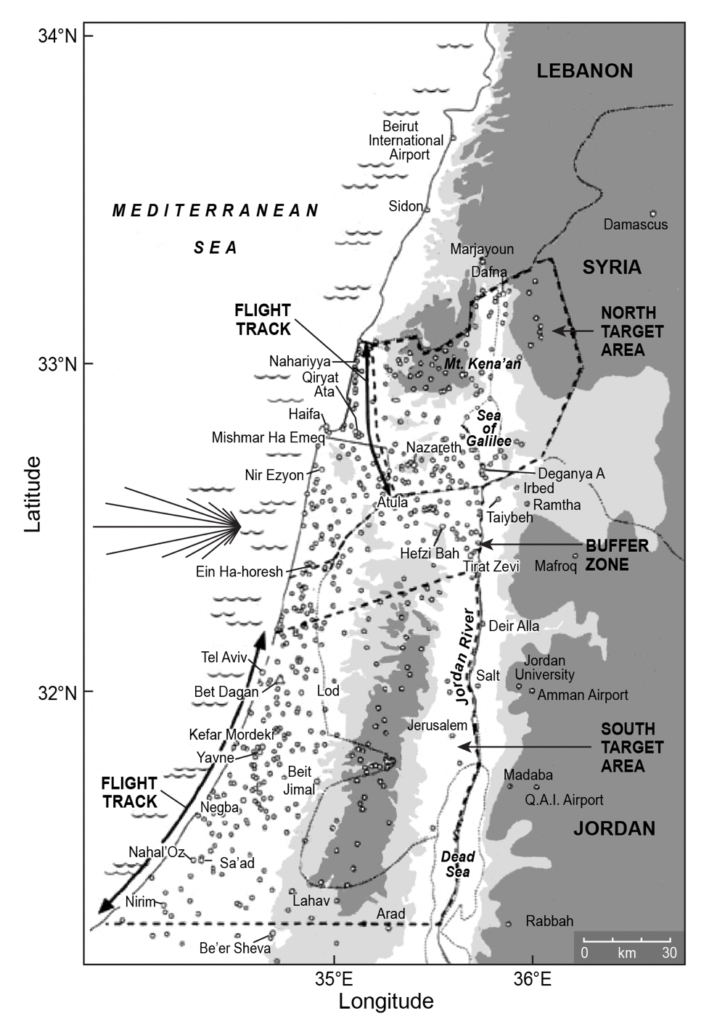
Figure 2. Map of Israel showing the two target areas and the buffer zone for Israel-2. The solid. lines with arrows denote the flight tracks along which artificial seeding was carried out. The circles show locations of IMS rain gauges. The triangle shows the location of the IMS 3-cm radar and rawinsonde launch site at Bet Dagan. The light shading shows terrain between 300 and 600 m MSL, and the darker shading terrain above 600 m MSL. The wind rose shows the per-centage of the time that the 850-hPa wind was from a particular direction when rain was falling at the time, or within 90 min, of the rawinsonde launch time and at, or within 60 km, of the rawinsonde launch site.
——————-
A narrow coastal region located upwind of the north target area, one that exhibited a high correlation (r≈0.9) in historical rainfall with the north target, was designated as a control area at least as early as 1972, adding another evaluation dimension for that target in addition to the primary crossover one (GN74a[11]).
The amount of seeding was significantly increased from Israel-1 to Israel-2. A second line-seeding aircraft was added, and a network of 42 generators was installed (National Academy of Sciences 1973, Appendix). The ground generators were added for more effective seeding of the inland hill regions of Israel than had been the case in Israel-1 where only four ground generators were used, and those were in the far northeast corner of the country (GN74a).
The Israel-2 experiment had several design/evaluation elements from which statistical results could be derived: a crossover design using the combined data from both targets; a target/control design for the north target; single area evaluations for each target using the rainfall on their respective seeded and unseeded days; and one using the alternate target’s rainfall on a target’s seeded day as the control rainfall. One of the key advantages of the crossover method, as was described in GN74a, is to reduce storm bias on experimental days when the alternate target’s non-seeded rainfall is used as a control.
The experimenters also had radar coverage during Israel-2 from the Israeli Meteorological Service’s (IMS) 3-cm wavelength radar from which to examine the echo tops of showers. The radar data were to prove critical in illuminating results of seeding on various categories of modal echo top temperatures in conjunction with IMS rawinsonde data.
d) The results of Israel-2
Preliminary results were reported for Israel-2 by GN74a and GN74b. GN74a reported that the target/control evaluation of the first two years had produced statistically-significant indications of rain increases in the north target of about 20% (single area ratio of 1.2) in the north target while in the south target using the same statistic was “less than 1,” suggesting rain had been decreased (GN74a). GN74a noted that cloud tops were appreciably lower and warmer by an average of about 4°C in the south target than in the north target, and that seeding was likely going to be less effective there due to those higher cloud top temperatures.
GN74b[12]reported the results of Israel-2 after three and four seasons, respectively. They reported results for the north target only, noting that rainfall in the south target was highly variable, and that it would take longer to determine any seeding results. The results of seeding in the north target remained virtually the same from season three to season four, with indicated rainfall increases of 13 and 14 %, respectively.
The greatest effect of seeding (statistically-significant) found by GN74b, was concentrated in the radar echo top temperature range of -15°C to -20°C, where prior calculations had suggested the greatest seeding effect should be contained given the belief that such clouds would be deficient in natural ice (e.g., Gagin and Steinhorn 1974).
The results of the completed Israel-2 experiment were first reported by GN76, and later by Gagin and Neumann (1981, hereafter, GN81); and in a series of reports by Gagin 1981; Gagin 1986, hereafter, G81 and G86 respectively), and also by Gagin and Gabriel 1987, hereafter GG87). All these reports that suggested a 13% increase in rainfall due to seeding were confined to the north target using only the target/control evaluation method. Larger increases were noted farther downwind from the cloud-base seeding line. The results of seeding using the other evaluation methodologies were not reported.
Radar top height measurements combined with rawinsonde data reinforced the earlier GN74b findings that the peak increase in rainfall on seeded days (46%) in the north target occurred when modal radar tops were between -16° and -21°C. Much smaller increases were indicated when modal tops were between -12°C and -16°C. No increases in rain were found for those tops outside of the -12° to -21°C partition. Radar top temperatures with seeding results were not reported for the south target.
Benjamini and Harpaz (1986) found evidence that the daily randomized experiment had increased runoff in streams and from springs over entire rain seasons. However, none were statistically significant. Ben-Zvi et al. (1987); Ben-Zvi (1988); and Ben-Zvi and Fanar (1996) followed with evaluations that found more robust indications of runoff and spring flow increases, some of which were statistically significant. Sharon (1990) combined the results of the studies mentioned above by grouping them all together and found still more statistically-significant runoff or flow increases from springs over whole rain seasons that he attributed to seeding. The increased spring emissions when compared to historical data (15 years prior to Israel-2) was about 10% and confined to a central target zone northeast of the aircraft line seeding path.
The results reported for Israel-2 north target appeared to offer an unambiguous confirmation of the rain increases due to seeding reported in Israel-1 for a wide scientific audience and as a stand-alone experiment (e.g., Tukey et al. 1978).
The first two Israeli experiments, as they had been reported, constituted formidable statistical evidence for a cloud seeding success in well-designed experiments having an exploratory phase followed by a confirmatory one. Now, with reports of rain increases confined to modal echo top temperatures that ranged from -12° to -21°C, the experiments seemed complete as an unambiguous testimony to the positive effect of random seeding with AgI.
4. The experimenters’ cloud microstructure reports
“The body of inferred physical evidence appears to support the claims of physical plausibility for-the positive statistical results of the replicated Israeli experiments I and·II (Gagin and Neumann, 1981) and helps to explain, in retrospect why these experiments were so successful, and others were not (Tukey et al., 1978; Kerr, 1982).”
——B. A. Silverman (1986)
A key ingredient buttressing the virtually unanimous acceptance of the statistical results of the Israeli experiments as proof of seeding efficacy were reports that the clouds of Israel were filled with great cloud seeding potential.
Why did the clouds of Israel appear so ripe for cloud seeding?
The experimenters reported on many occasions that ice crystal concentrations were quite low in Israeli clouds until their tops became colder than -21° C (e.g., Patrich and Gagin 1970; Gagin 1971; GN74a, b; Gagin 1975, hereafter G75, Gagin 1980, G81, G86. These reports meant that most of the cumuliform clouds rolled into Israel from the Mediterranean Sea with bases averaging 8°C-9°C, were 3-5 km deep with tops >-21°C produced little or no rain; that is, until they were seeded. According to these reports it took deeper, colder-topped natural clouds than these to produce significantnatural rain (e.g., G75; GN76; GG87). The lower part of this temperature range, from -16° to -21°C was also where the AgI used in Israel-2 was highly active in nucleating activity, another point adding credibility to the statistical results.
The wintertime cumuliform clouds of Israel were being described by the experimenters’ as mirror images of the microstructure that had been reported for the wintertime stratiform clouds in Colorado. In both locales it was reported that there was a relative dearth of ice crystals until cloud tops were colder than -21°C (e.g., Grant 1968; G75). Moreover, the reports from Colorado and Israel were also in agreement with summary reports like those of Fletcher (1962), Grant and Elliott (1974) and Cotton (1986) the latter two articles in particular purporting that a cloud top seeding “window” of opportunity existed for clouds with tops between -10°C and -25°C due to the belief that such supercooled clouds would be lacking in natural ice crystals. Thus, the Israeli experimenters’ reports of very low ice concentrations in clouds with tops >-21°C, and no ice in clouds with tops >-12°C to -14°C, fit the existing paradigm of ice in clouds and were widely accepted (e.g., Mossop 1985).
It was also reported by the experimenters, as it had been in Colorado, that the cloud seeding scourge of “ice multiplication” (Hobbs 1969, Dennis 1980) did not occur in Israeli clouds (e.g., G75; G81: G86; Figure 1]3, black dots).
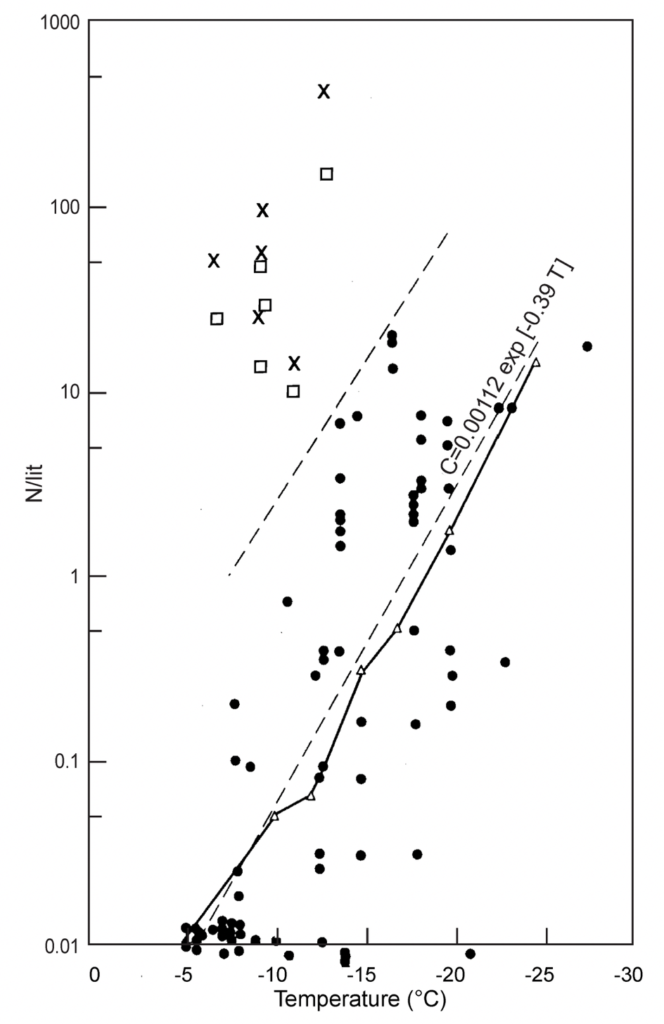
Figure 3.
Figure 3. Ice crystal concentrations vs. cloud top temperature (dots), including the least squares regression (dashed line) for these data (after Gagin 1975). In the original equation shown, the letter “C” denotes ice crystal concentration and the letter “T”, the cloud top temperature. The solid line with the open triangles denotes average ice nucleus spectrum. The “X’s” are ice crystal concentrations measured by Levin et al. (1996); the squares are one-half those values reported by Levin et al. (1996) to take in possible shattering artifacts. The upper dashed line represents a criteria suggested by Hobbs (1969) above which the observed concentrations of ice crystals qualify as a case of “ice multiplication.”
==================================================
“Ice multiplication” is where 100’s to 1000’s more ice crystals are found in clouds than can be accounted for by standard ice nuclei concentrations as summarized by Fletcher (1962) from world studies. Ice multiplication is thought to severely reduce or eliminate cloud seeding potential in the kind of cloud seeding experiments carried out in Israel where small amounts of AgI are released (e.g., Dennis 1980).
In both the exact temperature range in which seeding appeared to have produced the greatest results, and in the magnitude of the response to seeding (about 50% increases in precipitation), Israel-2 was also a mirror image of the results of cloud seeding that had been reported by Colorado scientists (e.g., Mielke et al. 1970; 1971; 1981). It was a remarkable confluence of reports considering the different types of cloud systems seeded (cold stratiform vs. cumuliform).
Further parallels in these sets of experiments in Colorado and Israel-2 which added to the credibility of both, were that no viable seeding effects occurred at “cloud top” temperatures above -12° C or below -21°C. The high temperature cutoff was attributed to the low nucleating activity of the silver iodide nuclei used to seed their respective clouds, low crystal growth rates, and the shallowness of clouds having those warmer tops. The low temperature cutoff of seeding effects was thought to be due to the high natural concentrations of ice believed to exist at cloud top temperatures <-21°C where AgI would have little impact (amplifying the importance of not having ice multiplication occur).
The final parallel reported between the experiments in Colorado and Israel, and one that was also critical to the credibility of the statistical results, was that the effect of seeding had been to create more hours of precipitation; it had not increased its intensity (e.g., Chappell et al. 1971, in Colorado; G86; GG87 in Israel-2)[1]. The duration finding was again compatible with the kind of seeding carried out in each experiment, termed, “static seeding”, where relatively low concentrations of AgI are released into the clouds to initiate precipitation that otherwise would not have occurred (Dennis 1980; National Academy of Sciences 2003).
Thus, in every way, despite the differences in the clouds seeded, the reports from the experiments in Colorado and in Israel “cross pollinated” one another, helping to increase their mutual credibility.
5. The Fall
a. The erosion of the experimenters’ cloud reports, and ultimately, the foundation for the belief that cloud seeding had increased rainfall
Reversals of the descriptions of the “ripe-for-seeding” Israeli clouds began to appear in 1988 when it was reported that rain fell from clouds with tops >-10°C (R88), contrary to the experimenters’ many cloud reports. In six flights on shower days a few years later by Levin (1992; 1994; Levin et al 1996, Table 4), high concentrations (10’s to 100’s per liter) of ice particles were encountered near the tops of clouds ranging from just -6°C to -13°C (Figure 3, crosses). The values reported by Levin et al. (1996) indicated that ice multiplication is rampant in Israeli clouds and supported the conclusions in R88.[2] Radar observations by Rosenfeld and Gagin (1989) showed that rain initiates in Israeli cumuliform clouds between -5°C and -10°C when temperatures, estimated by using pseudo-adiabatic lapse rates, are added to their Figure 1. This is precisely what was reported in a similar figure using the IMS radar by GN74 but went unnoticed.
Ramanathan et al. (2001) using satellite-derived effective radius measurements confirmed the above results that precipitation onset in Israeli clouds with tops between -5°C and -8°C, typically when cloud depth is between 2.5 and 3.5 km. Ramanthan et al. however, attributed their finding to the presence of dust aerosols rather than reporting that it was a general characteristic of Israeli clouds. That was to be found later.
Freud et al. 2015, however, attributed the early formation of precipitation in Israeli clouds that they observed in tops ascending through -3°C to -5°C, to a “sea spray cleansing” of pollution aerosols from clouds by the Mediterranean Sea. This “cleansing” they asserted, allowed large droplets to form in clouds as they moved over the Mediterranean Sea toward and into Israel. The presence of large droplets made them conducive to early precipitation formation (and ice multiplication), “even before AgI can take effect,” Freud et al. wrote.
The early idea Israeli clouds are solelycontinental in character as had been reported by the original experimenters, that is, that they are characterized by very high droplet concentrations (500-1500 cm-3) and a narrow droplet spectrum throughout, has been revised due to these later reports. Cloud droplet concentrations in Israeli clouds are only moderately high, from 200-500 cm-3, or “semi-continental,” with exceptions that concern shallow boundary layer clouds in light winds in polluted conditions, almost always on non-storm days.
When these new reports of ice and precipitation onset in Israeli clouds are integrated into the nomogram of Rangno and Hobbs (1988, Figure 3[3]), the clouds of Israel are compatible in the onset of ice to similar clouds having similar base temperatures. They no longer standout as having to be significantly colder than similar clouds as had been indicated in the early cloud studies by the experimenters (e.g., G75). Moreover, with the lower droplet concentrations (e.g., Levin et al. 1996) and with cloud base temperatures averaging 8°C to 9°C, (GN74, G75), the Israeli clouds now also fall where Mossop’s (1978) nomogram predicts that ice multiplication will occur.
As with other clouds, dust is not required for enhanced ice concentrations; rather, just a broad droplet spectrum is required for Cumulus cloud tops that ascend to below freezing temperatures. With their relatively warm cloud bases, ranging from 5°C to 12°C (RH95a), modest droplet concentrations and a broad droplet spectrum, the clouds of Israel are indeed, “ripe”, but not for cloud seeding, but rather for ice multiplication and high ice particle concentrations, conditions that make them unsuitable for cloud seeding.[4]
b. The effectiveness of airborne line cloud seeding in Israel-1 and Israel-2
The line-seeding by a single aircraft used in Israel-1 was evaluated in RH95a. They concluded that the line-seeding method only could have affected a small fraction of all those that produce rain in Israel. It should be emphasized that the aircraft did not “orbit” in updrafts under promising cloud bases, but merely flew in a line under clouds and showers, whatever their stages (growing or dissipating), and in the clear spaces between them, releasing AgI nuclei all the while. The long seeding path resulted in untreated clouds going by before the seeding aircraft could return to its starting point.
Levin et al. (1997) also evaluated the aircraft line-seeding method, but for Israel-2, using the Colorado State University RAMS model with a 0.5 km mesh. They concluded, using rawinsonde profiles on typical shower days in Israel to initialize the model, that the aircraft line-seeding method could only have affected a very few clouds under which the aircraft flew. Their conclusion, using much more sophisticated calculations than in RH95a, was virtually identical to it.
c. The erosion of the statistical results of the first two Israeli cloud seeding experiments.
“Experiments with ‘unsuccessful’ results in the first season or two may often not be reported at all. As a result the experiments whose results are published would be those with initial ‘successes’ which are usually followed, sooner or later, by less ‘successful’ seasons.” —–K. R. Gabriel (1967a), quoting a personal communication from fellow statistician, W. Kruskal.
-
-
- Israel-1
-
Re-analyses of both Israel-1 and Israel-2 were carried out by RH95a. Considerable evidence was found for Type I statistical errors or “lucky draws” in each experiment[5]. Ironically, the RH95a findings for Israel-1 and Israel-2 replicated what had happened in the Climax I and II experiments where each of those experiments was found to have suffered from “lucky draws” (Mielke 1979), thus continuing a theme of these experiments mirroring each other’s experimental sequence of events in an unexpected way.
For Israel-1, Brier et al. (1974) had earlier interpreted regional cloud seeding statistics in Lebanon and Jordan as evidence of massive downwind and side wind seeding effects on center and north target seeded days. RH95a, on the other hand, saw the Brier et al. plots and statistics, in view of how little seeding that was carried out in Israel-1 in largely unsuitable-for-seeding clouds, as equally massive counter evidence of a bias in the random draws. RH95a also calculated that it was untenable that the small amount of AgI released in Israel-1 created the amount of rain over the vast area that Brier et al. had concluded that rain had been increased.
Sharon’s (1978) study that indicated that rainfall areas were larger on seeded days than on control days can be seen as compatible with a Type I statistical error or a “lucky draw.” Stronger storms on seeded days, rather than seeding effects, are more likely to have produced the larger areas of rain that Sharon (1978) attributed to seeding.
Another “red flag” in Israel-1 indicating something was likely amiss was that the little seeded BZ between the “center” and the “north” targets exhibited the greatest statistical significance of either north or center targets (e.g., Wurtele 1971; GN74a). As noted, the Chief Meteorologist of the Israeli experiments, (quoted by Wurtele 1971) in his own wind analysis, concluded that the BZ could only have been inadvertently seeded a miniscule amount of the time that seeding took place.
RH95a in their own low-level wind analysis reached a conclusion similar to that of the “Chief Meteorologist” quoted by Wurtele. RH95a focused on those times when rain was falling in Israel at within 90 min of the time of the Bet Dagan, Israel, rawinsonde launch, replicating those times when seeding would have been expected to be taking place. The very narrow wind direction envelope with rain falling (see wind rose in Figure 1) suggests that it would have taken a fairly negligent pilot to have inadvertently seeded the BZ on center-seeded days if he had been instructed not to seed it.
Adding to this picture of an uneven draw that favored heavier natural rain in the BZ on center seeded days was greater rainfall on those same days at coastline locations too close to have been affected by a fallout of rain from cloud base seeding (RH95a). This same conclusion about the coastal zone having been unaffected by seeding had been reached earlier on several occasions by the experimenters (Neumann et al. 1967 N[6]; Gabriel 1967a; Gabriel and Baras 1970 N; Gabriel 1979).
Thus, the totality of evidence above for Israel-1 best supports the “lucky draw” hypothesis (Type I statistical error) that created the misperception of increased rainfall due to seeding. This conclusion is compatible with too little seeding in Israel-1 and one also compatible with today’s knowledge that the clouds of Israel are unreceptive to producing significant rain through glaciogenic seeding with AgI (e.g., R88, Levin et al. 1996).
Moreover, the findings of Freud et al. (2015) have undercut the multi-faceted hypothesis of Rosenfeld (1997) who tried to the explain the high seed/no seed ratios in the immediate coastal zone of Israel-1 as due to a “blowback” of cloud seeding material released by the seeding aircraft. Rosenfeld posited that a portion of the seeding material released in westerly or southwesterly flow at cloud base offshore eventually dispersed downward, got caught in low-level, offshore-flowing easterlies near the surface as the AgI diffused downward and eastward into Israel. At that point a portion of the seeding plume reversed course and headed to the west or northwest. It not only went offshore, he surmised, but offshore far enough that it got ingested into the bases of ripe-for-seeding clouds at locations far enough upwind so that when the AgI rose up the several km required to where the errant AgI could activate, it then triggered ice crystals that grew and fell out as rain on the coastal zone. These many-linked conjectures “explained” the high seed/no seed ratios on seeded days along the coast according to Rosenfeld (1997).
The ripe-for-seeding clouds hypothesized by Rosenfeld (1997), ones awaiting the errant seeding plume moving westward and offshore, however, have been shown to be mirages in the many cloud-related citations above, among other unrealistic aspects of this hypothesis critical of the RH95a reanalysis. Rosenfeld’s long 1997 commentary was comprehensively addressed in RH97b.
-
-
- Israel-2
-
The convincing results for the north target of Israel-2 with so many supporting arguments, were compromised when the full results of the experiment were reported (Gabriel and Rosenfeld 1990). Gabriel and Rosenfeld found that the mandated crossover analysis of Israel-2 resulted in no apparent seeding effect (-2%), reversing the former “optimistic results” of seeding, they wrote.
The major culprit?
Unusually heavy rain on north target seeded days also fell in the unseeded south target, the north’s control area in the crossover design. How heavy were those rains in the south target on north target seeded days?
Quoting Gabriel and Rosenfeld (1990) on their extraordinary discovery in this regard: the south target rainfall was “several standard errors above the normal daily amount” and it was “clearly statistically significant[7].” Any real seeding effect in the north may have been canceled out in a crossover type of evaluation. Gabriel and Rosenfeld (1990) were not able to clarify whether there had been real increases in rain the north target area (13%) and decreases in the south (-15% or more), as their results suggested, or whether there had been no seeding effects at all in both targets.
However, the statistically significant results using one of the several evaluation methods, the target/control scheme, held out hope that cloud seeding had nevertheless increased rainfall.
Rosenfeld and Farbstein (1992, hereafter, RF92) capitalized on the possibility of actual “divergent” effects due to seeding suggested by Gabriel and Rosenfeld (1990). They hypothesized that the increases and decreases suggested in rainfall due to seeding in the two targets were real and were were due to the presence or absence of dust. Surface weather observations for the presence of dust or haze were examined by RF92 and those days where one or more Israeli surface stations reported “dust-haze” were separated from days without dust-haze and the results of seeding re-evaluated. The elimination of numerous “dust-haze” days led to improved seeding results in the north target (RF92).
There were several assumptions in the dust-haze hypothesis of RF92: 1) a report of dust-haze at the ground meant that the clouds aloft had been seriously impacted by dust-haze, 2) the kind of dust that the clouds ingested led to large cloud droplets, 3) the large cloud droplets led to both the formation of rain through an all liquid process (collisions of droplets with coalescence), and, if cool enough at cloud top, to high ice particle concentrations.
They further hypothesized that when seeded, such clouds affected by dust-haze developed too many natural ice crystals for effective rain at the ground. The more numerous, smaller ice crystals in clouds due to seeding with dust in them resulted in less rain at the ground because the smaller, more numerous ice crystals evaporated on the way down before they could become raindrops.
In this “divergent effects” hypothesis by RF92, it was recognized that most of the clouds of Israel have naturally high ice particle concentrations, but solely due to dust-haze, except for a portion of those clouds which, by inference, RF92 still deemed as “ripe-for-seeding” when dust-haze was not affecting them. RF92’s findings also meant that the clouds of Israel generally did not contain modest droplet concentrations with a broad droplet spectrum without dust. The latter combination would stillmake such clouds ready to produce high ice particle concentrations at slight to modest supercooled cloud top temperatures and unsuitable for producing appreciable results from cloud seeding. There were no in-cloud measurements to support the RF92 hypothesis concerning the effect of dust beyond ground ice nuclei measurements (Gagin 1965) and soil particles in rainwater (Levi and Rosenfeld 1996).
RH95a, inspired by the RF92 re-analysis of Israel-2 and the “dust-haze” hypothesis, carried out another, but wider re-analysis of Israel-2, one that incorporated data from Lebanon[8] and Jordan. RH95a concluded that a Type 1 statistical error (lucky draw) had occurred in Israel-2 north target seeded days and that it had produced the misperceptions of increased rain in the north target area (Type I statistical error, or “lucky draw”) and one of decreased rain in the south target area (Type II statistical error, or “unlucky draw”.) Namely, there were no “divergent” effects of seeding, as hypothesized by RF92. The RH95a conclusion was reached because not only did the south target experience unusually heavy rain on north target seeded days (the south’s control day for the single area seed/no seed ratio), but sites in Lebanon and Jordan also experienced heavier rain on north target seeded days. Thus, rain wasn’t decreased on south target seeded days as hypothesized by RF92, but rather excessive rain on the south’s control days produced an appearance of decreases due to seeding when only average rain fell on its seeded days.
Furthermore, since cloud tops are warmer and lower as a rule in the South target in Israel than in northern Israel (GN74a; RH95a) it is difficult to accept the proposition by RF92, and later by Rosenfeld and Nirel (1996), that clouds in southern Israel could have been “overseeded” due to dust combined with AgI.
It was also observed in the wider analysis by RH95a that the rain gauges used by the experimenters in the small coastal control zone as a control for the north target constituted an anomaly in the regional pattern of heavier rainfall on the north target’s seeded days. The narrow coastal control zone did not reflect the regionally wide heavier rainfall. This enigma was not resolved by RH95a but was resolved later by Levin et al. (2010).
Levin et al. (2010) addressed the question of synoptic bias in Israel-2 and found that synoptic factors had, indeed, compromised Israel-2. Stronger upper low centers were in the eastern Mediterranean accompanied by stronger low-level winds on the north target’s seeded days. These stronger storms “drove” the Israel-2 statistical results when the coastal control zone was used. The stronger lower level winds created a pseudo-seeding effect by intensifying the maximum rainfall from the coastal control zone toward the hilly regions of the target, the rain amplified by orographic effects. Under stronger onshore winds, the coastal convergence zone that leads to heavy coastal rains is not active.
The re-analyses by Levin et al. (2010) of the Israel-2 north target and of operational seeding, as did that of RH95a, drew vigorous commentary from seeding partisans (Ben-Zvi et al. 2011), with a comprehensive “Reply” by Levin et al. (2011). The INWA was not inspired to resume operational seeding based on the arguments of Ben-Zvi et al. 2011. Instead, the INWA moved on to a new experiment, Israel-4, to test whether cloud seeding can increase rainfall in Israel. The results of this experiment are discussed later.
3. The Israel-3 randomized experiment; the longest, least known cloud seeding experiment ever carried out.
While operational seeding began in northern Israel in 1975[9]triggered by reports of rain increases due to seeding in Israel-2 for its north target (GN76), a new daily randomized seeding experiment, called Israel-3, began in an expanded region of the former south target of Israel-2. This larger target required a longer line-seeding path by the aircraft. Changes in the ground seeding network in Israel-3 from Israel-2, if any, have not been reported.
The results of this experiment began to appear in the literature in 1992, 17 years after it began in RF92[10]. RF92 reported that there was a non-statistically significant indication that rain had been decreased by about 8% due to cloud seeding. A similar interim report was presented by Nirel and Rosenfeld (1994). The final result of cloud seeding in Israeli 3 was reported at conference by Rosenfeld (1998). After 20 winter seasons and 936 daily random decisions, there was an indication of a 9% decreasein rainfall (non-statistically significant) due to seeding. Several exploratory analyses were put forward by Rosenfeld (1998), however, that suggested might have been increased in some situations.
The suggestion of appreciable decreases in rain on seeded days in Israel-3 constituted a discouraging blow to the daily randomization of cloud seeding experiments as did Israel-2. It would not be expected in an experiment of so many daily randomizations over 20 winter seasons, with no effect on rainfall due to seeding (as concluded by Rosenfeld 1998), that a statistical result could drift as far as -9% from an expected null result. An unbiased random draw of rain days would have been expected to have produced a result near zero indicated effect.
There are four major conclusions that can be drawn from Israel-3: 1) the result corroborates the lack of increased rain due to seeding in Israel-1 and Israel-2; 2) the results of all of these experiments, en toto, might be ascribed to a poor seeding methodology that led to ineffective coverage and cloud treatment; 3) dailyrandomization has not proved to be the panacea leading to unbiased natural storm distributions in cloud seeding experiments that it was hoped to be; 4) and probably the most important factor impacting all of these results; the clouds of Israel are, overall, unreceptive for the production of meaningful increases in rain through AgI seeding due to their naturally high precipitating efficiency and readiness for early natural ice formation at slightly supercooled temperatures.
6. Evaluations of operational cloud seeding, 1975-2002.
Due to the RH95 re-analyses of the Israeli cloud seeding experiments, the ensuing exhaustive commentaries and replies in 1997, and Levin et al.’s 1997 modeling study that indicated airborne seeding at cloud base was ineffective, the Israel National Water Authority (INWA) formed an independent panel of experts to evaluate the results of operational seeding to increase runoff into Lake Kinneret (the Sea of Galilee). The final evaluation by Kessler et al. (2006, in Hebrew with an English abstract), distilled by Sharon et al. (2008), did not find evidence that cloud seeding had been increasing runoff (Figure 4).
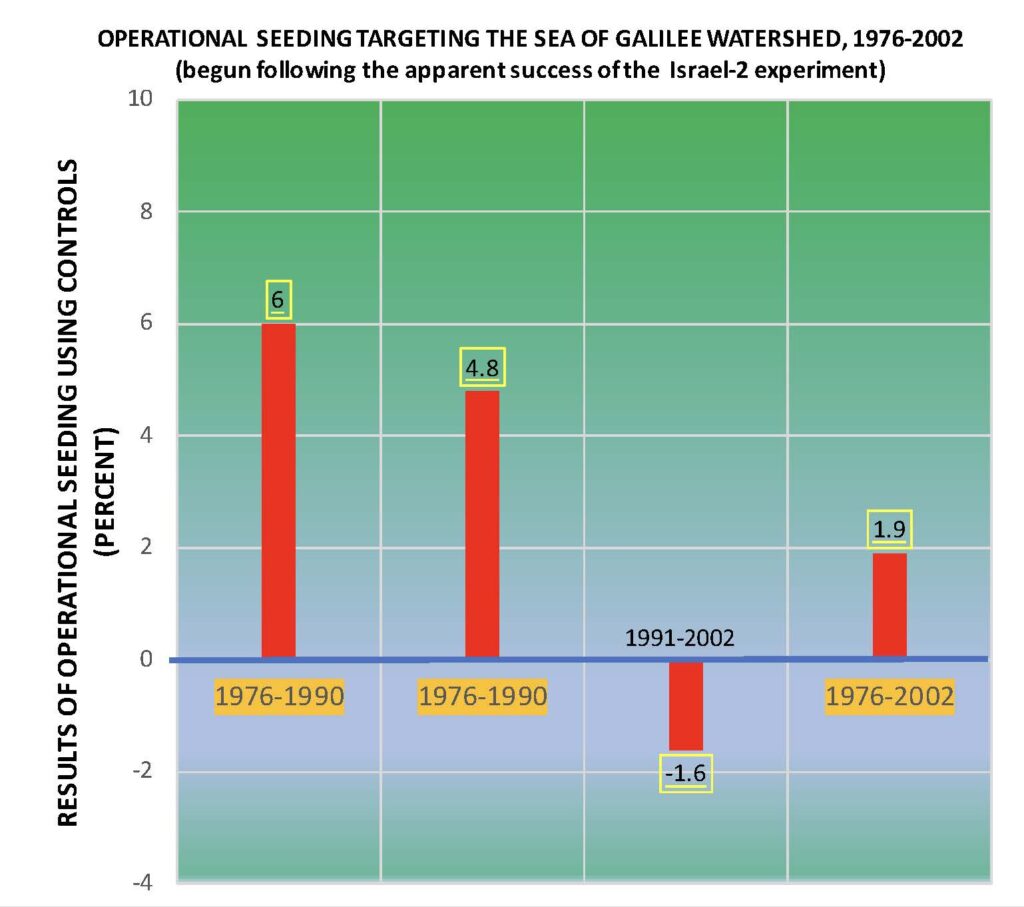
Figure 4. The results of the independent evaluation of operational cloud seeding on rainfall in the Sea of Galilee watershed by Kessler et al. (2006) are shown by the rightmost three columns for the periods shown. The Hebrew University of Jerusalem evaluation published by Nirel and Rosenfeld (1995) is the leftmost column.
Kessler et al’s result was contrary to seeding expectations based on many earlier reports suggesting runoff increases in streams and springs over whole seeding seasons (Benjamini and Harpaz 1986; Ben-Zvi et al. 1987; 1988; Ben-Zvi and Fanhar (1996); Sharon 1990; and in an updated report on operational seeding results through 1990 by Nirel and Rosenfeld (1995). A second independent analysis of the operational seeding program by Levin et al. 2010 corroborated the findings of Kessler et al (2006) and Sharon et al. (2008).
Due to the findings in Kessler (2006), operational seeding, in Israel was terminated at the end of the 2007 winter season (Sharon et al. 2008). These results meant that millions of dollars might have been wasted on operational cloud seeding in Israel for over 30 years, findings that weighed heavily on the HUJ experimenters whose work the operational seeding had begun under. This was not to go unchallenged.
The first HUJ response to interim findings of Kessler et al (2002) of no seeding results was by Givati and Rosenfeld (2005). While agreeing that no additional runoff due to seeding was occurring in the operational seeding program after 1990, they argued that air pollution was masking seeding increases in rain. In fact, they claimed, it was decreasing rain exactly as much seeding was increasing it, leading to a null seeding result in rainfall.
The air pollution claims, while superficially credible except for their sudden hypothesized appearance, were evaluated by several independent groups and scientists besides Kessler et al. (2006) who did not find them credible: Alpert et al. (2008); Halfon et al. (2009); Levin 2009; and addressed in a review by Ayers and Levin (2009). All these independent re-analyses and reviews of the hypothesized effect of air pollution on rainfall found the argument that air pollution had canceled seeding-induced increases in rain unconvincing.
Givati and Rosenfeld (2009) contested the findings of Alpert et al. 2008 and submitted a wider analysis that used more gauges than they had previously. Alpert et al. (2009) responded to the new data presented by Givati and Rosenfeld (2009) showing that the new data of Givati and Rosenfeld (2009) had inadvertently strengthened the original Alpert et al. (2008) conclusions.
The bottom line was that rain gauges could be found that could support either a pollution effect or a no effect of pollution claim, thus it was not a robust claim having much veracity. Thom (1957) first noted that virtually any result can be found via cherry-picking of control gauges amid many candidates to prove a seeding effect. There are more than 500 standard gauges and 82 recording gauges in Israel from which to extract seeding effects (A. Vardi, Deputy Director, the IMS, 1987, private correspondence).
More than any words here can demonstrate, it was the INWA’s decision to terminate and not resume operational seeding of the Sea of Galilee catchment that was the final arbiter in settling which of the above arguments were the most convincing to them, the funder of cloud seeding activities.
7. About Israel-4
The INWA began a new, long-term randomized cloud seeding experiment in the 2012-2013 rain season in the Golan Heights, termed Israel-4. The experiment was based on the findings of 27 research flights carried out by the HUJ in the search for the best location in Israel to have the best chance of proving that cloud seeding can add measurably to Israel’s water needs. The results of these flights, summarized by Freud et al. 2015, was that the region of the Golan Heights would make the best site for a new cloud seeding experiment based on airborne observations of “abundant” supercooled water. The experiment concluded after seven seasons of random seeding in 2020 with a null result, a suggested non-viable 1.8% increase in rain (Benjamini et al. 2023). So far, the INWA has decided not to pursue further cloud seeding based on this result.
It is noteworthy that Israel-4 was not conducted by the HUJ scientists, but rather by a collection of other independent Israeli scientists, statisticians, and hydrologists. Whether this result will be challenged by HUJ scientists has yet to be determined, but one would expect a challenged based on the past.
- Summary
The reader may wonder at this point how so many flawed cloud reports and only the partial statistical results of a major, benchmark cloud seeding experiment could be cleared for the peer-reviewed literature, literature that led to a scientific consensus that cloud seeding had been proved in Israel–a consensus that affected a wide range of stakeholders, including Israel’s own government?
There is a multi-pronged answer to this question: 1) the cloak of daily randomization likely misled experimenters who expected a neutral random draw, considering the length of the Israeli experiments and dismissed the possibilities of natural bias; 2) inadequate and/or conflicted (“friendly”) peer reviews of manuscripts that, in retrospect, demanded too little of the experimenters; 3) a lack of full reporting of experimental results by the experimenters (i. e., all of Israel-2 when it was concluded, and those from Israel-3 in a “timely manner” as suggested by the AMS in its “Guidelines” for Professional Conduct[11]).
But perhaps the most important element of all, was the experimenters’ failure to discern the natural character of their efficiently precipitating clouds which ultimately cost them and the Israeli people so much. Moreover, the original experimenters rebuffed independent airborne research efforts to measure the interesting properties of their clouds over the years (G. Vali, personal communication, 30 January 1986)[12].
Why?
It’s clear that outside researchers would have quickly discovered the true nature of Israeli clouds and informed the world and the HUJ experimenters about them.
And why did it take the HUJ experimenters 35 years after they monitored their clouds with two radars, one that was vertically pointed and over flown by their aircraft to validate cloud tops (G80, Rosenfeld 1980) to discover that the clouds entering Israel had been “sea spray cleansed” and formed precipitation at modest cloud top heights and temperatures as was finally reported by Freud et al. 2015?
Too, the absence of efforts by the original experimenters to examine the natural weather patterns, uneven storm draws, leaving it to outsiders, speaks volumes to entrenched confirmation and desirability biases.
8. Reflections on the rise and fall of Israeli cloud seeding.
Given this account, one cannot help but ask if the full results of Israel-2 had been reported in a timely manner, as well as those from Israel-3, as it proceeded, and if the experimenters had gotten the Israeli cloud microstructure correct from the outset, would the Israeli government have pursued operational seeding of the Sea of Galilee watershed with no viable result at a cost of $20 million or more over the 32 years following the conclusion of Israel-2?
It is also evident that it is unwise to have the same scientists who carried out a seeding experiment, or personnel within their home institutions, evaluate its results or report on the potential of clouds for seeding purposes. Independent evaluations by those not having vested interests (operational or otherwise) in cloud seeding should have beenmandatory. The Israeli’s showed us the way with the INWA’s brave move to have an independent panel of experts evaluate their long-term operational cloud seeding effort.
Moreover, the HUJ cloud seeding experimenters have been stymied for more than 25 years in their airborne most recent efforts to measure a critical parameter necessary to fully evaluate the seeding potential of their clouds: ice particle concentrations and the rapidity of their development.
It is urgent for the people of Israel and the INWA that extensive, independent airborne measurements of Israeli clouds carried out soon by groups not relying on cloud seeding funding, and whose aircraft instrumentation can measure ice particle concentrations reliably in Israeli clouds.
Acknowledgements. Thanks to Prof. Bart Geerts for his many suggestions on an early draft. I also thank environmental writer, Maria Mudd Ruth, for an encouraging assessment of an early draft. I thank Prof. David Schultz for a late, highly valuable review. I also thank the two official reviewers, Dr. Daniel Rosenfeld (the “reject” reviewer) and the anonymous Reviewer 2 (“important paper, accept, minor revisions”) for their many insights that resulted in some corrections. Figures 1-3 were improved from original versions by Tully Graphics.
Author disclosure: I have no funding sources but my own. I have worked on both sides of the seeding “fence”; in operational seeding programs in South Dakota (twice), in the Sierras, in Washington State, and in India. I have participated in seeding research at the University of Washington and with the National Center for Atmospheric Research in Saudi Arabia. I was the Assistant Project Forecaster with the Colorado River Basin Pilot Project, a large randomized orographic cloud seeding experiment, 1970-1975.
REFERENCES
Alpert, P., N. Halfon, and Z. Levin, 2008: Does air pollution really suppress precipitation in Israel? J. Appl. Meteor. Climatology, 47, 943-948. https://doi.org/10.1175/2007JAMC1803.1
_______, __________, _________, 2009: Reply to Givati and Rosenfeld. J. Appl. Meteor. Climatology, 48, 1751-1754. https://doi.org/10.1175/2009JAMC1943.1
Ayers, G., and Z. Levin, 2009: Air pollution and precipitation. In Clouds in the Perturbed Climate System. Their Relationship to Energy Balance, Atmospheric Dynamics, and Precipitation.J. Heintzenberg and R. J. Charlson, Eds. MIT Press, 369-399. No doi.
American Meteorological Society, 1984: Statement on Planned and Inadvertent Weather Modification. Bull. Amer. Meteor. Soc.,66, 447-448. No doi.
Ben-Zvi, A., 1988: Enhancement of runoff from a small watershedby cloud seeding. J. Hydro!. 101, 291-303. No doi.
________, 1997: Comments on “A new look at the Israeli randomized cloud seeding experiments.” J. Appl. Meteor., 36, 255-256.
https://doi.org/10.1175/1520-0450(1997)036%3C0255:COANLA%3E2.0.CO;2
________, and A. Fanar, 1996: Effect of cloud seeding on rainfall intensities in Israel. Isr. J. Earth Sci., 45, 39-54. No doi.
________, Rosenfeld, D., A. Givati, 2010. Comments on “Reassessment of rain experiments and operations in Israel including synoptic considerations” by Levin, N. Halfon and P. Alpert, Atmos. Res., 97, 513-525. https://doi.org/10.1016/j.atmosres.2010.06.011
________, S. Massoth, and B. Anderman, 1987: Changes in springflow following rainfall enhancement. Isr. J. Earth Sci., 37, 161-172. No doi.
Benjamini, Y., and Y. Harpaz, 1986: Observational rainfall-runoff analysis for estimating effects of cloud seeding on water resources in northern Israel. J. Hydrol., 83, 299-306. No doi.
Braham, R. R., Jr.., 1986: Precipitation enhancement–a scientific challenge. In Precipitation Enhancement–A Scientific Challenge, R. R. Braham, ed., Meteor. Monog. 21, No. 43, 1-5. https://doi.org/10.1175/0065-9401-21.43.1
Brier, G. W., L. O. Grant, and P. W. Mielke, Jr., 1974: An evaluation of extended area effects from attempts to modify local clouds and cloud systems. Proc., WMO/IAMAP Scien. Conf. on Weather Modification, Tashkent, World Meteor. Org., 439-447. No doi.
Broad, W. J., and N. Wade, 1982: Betrayers of the Truth: Fraud and Deceit in the Halls of Science. Simon and Schuster, 256pp. No doi.
Bruintjes, R. T, 1999: A review of cloud seeding experiments to enhance precipitation and some new prospects. Bull. Amer. Meteor. Soc., 80, 805-820. https://doi.org/10.1175/1520-0477(1999)080%3C0805:AROCSE%3E2.0.CO;2
Changnon, S. A., and W. H. Lambright, 1990: Experimentation involving controversial scientific and technological issues: weather modification as a case illustration. Bull. Amer. Meteor. Soc., 71, 334-344. https://doi.org/10.1175/1520-0477(1990)071%3C0334:EICSAT%3E2.0.CO;2
Chappell, C. F., L. O. Grant, and P. W. Mielke, Jr., 1971: Cloud seeding effects on precipitation intensity and duration of wintertime orographic clouds. J. Appl. Meteor., 10, 1006-1010.https://doi.org/10.1175/1520-0450(1971)010%3C1006:CSEOPI%3E2.0.CO;2
Cotton, W. R., 1986: Testing, implementation, and evolution of seeding concepts–a review. In Precipitation Enhancement–A Scientific Challenge, R. R. Braham, Jr., Ed., Meteor. Monographs, 21, No. 43, Amer. Meteor. Soc., 139-149. https://doi.org/10.1175/0065-9401-21.43.139
___________., and R. A. Pielke, 1995: Human Impacts on Weather and Climate, 2nd edition, Cambridge University Press, 288pp. No doi.
Dennis, A. S., 1980: Weather Modification by Cloud Seeding. Academic Press, NY, 145. No doi.
__________, 1989: Editorial to the A. Gagin Memorial Issue of the J. Appl. Meteor., 28, 1013. No doi.
__________, and H. D. Orville, 1997: Comments on “A new look at the Israeli cloud seeding experiments.” J. Appl. Meteor., 36, 277-278. https://doi.org/10.1175/1520-0450(1997)036%3C0277:COANLA%3E2.0.CO;2
Fletcher, N. H., 1962: The Physics of Rainclouds. Cambridge University Press, 242pp. No doi.
Freud, E., H. Koussevitsky, T. Goren and D. Rosenfeld, 2015: Cloud microphysical background for the Israeli-4 cloud seeding experiment. Atmos. Res., 158-159, 122-138. http://dx.doi.org/10.1016/j.atmosres.2015.02.007
Gabriel, K. R., 1967a: The Israeli artificial rainfall stimulation experiment: statistical evaluation for the period 1961-1965. Vol. V., Proc. Fifth Berkeley Symp. on Mathematical Statistics and Probability, L. M. Le Cam and J. Neyman, eds., University of California Press, 91-113. No doi.
___________, 1967b: Recent results of the Israeli artificial rainfall stimulation experiment. J. Appl. Meteor., 6, 437-438. https://doi.org/10.1175/1520-0450(1967)006%3C0437:RROTIA%3E2.0.CO;2
____________, 1979: Comment. J. Amer. Statist. Assoc., 74, 81-84.https://doi.org/10.2307/2286727
___________, 1999: Ratio statistics in weather modification experiments. J. Appl. Meteor., 38, 290-301. https://doi.org/10.1175/1520-0450(1999)038%3C0290:RSFREI%3E2.0.CO;2
___________., and M. Baras, 1970: The Israeli rainmaking experiment 1961-1967 Final statistical tables and evaluation. Tech. Rep., Hebrew University, Jerusalem, 47pp. No doi.
___________., and J. Neumann, 1978: A note of explanation on the 1961–67 Israeli rainfall stimulation experiment. J. Appl. Meteor., 17, 552–556.https://doi.org/10.1175/1520-0450(1978)017%3C0552:ANOEOT%3E2.0.CO;2
___________, and Rosenfeld, D., 1990: The second Israeli rainfall stimulation experiment: analysis of precipitation on both targets. J. Appl. Meteor., 29, 1055-1067. https://doi.org/10.1175/1520-0450(1990)029%3C1055:TSIRSE%3E2.0.CO;2
Gagin, A., 1965: Ice nuclei, their physical characteristics and possible effect on precipitation initiation. Preprints, International Cloud Physics Conf., Sapporo. No doi.
_______, 1971: Studies of the factors governing the colloidal stability of continental clouds. Proc., Intern. Conf. on Weather Modification, Canberra, Amer. Meteor. Soc., 5-11. No doi.
_______., 1975: The ice phase in winter continental cumulus clouds. J. Atmos. Sci.,32, 1604-1614. https://doi.org/10.1175/1520-0469(1975)032%3C1604:TIPIWC%3E2.0.CO;2
_______., 1980: The relationship between depth of cumuliform clouds and their raindrop characteristics. J. Rech. Atmos., 14, 409-422. No doi.
_______., 1981: The Israeli rainfall enhancement experiments. A physical overview. J. Wea. Mod., 13, 108-122. No doi.
_______., 1986: Evaluation of “static” and “dynamic” seeding concepts through analyses of Israeli II and FACE-2 experiments. In Precipitation Enhancement–A Scientific Challenge, Meteor. Monog., 21, No. 43, Amer. Meteor. Soc., 63-70. https://doi.org/10.1175/0065-9401-21.43.63
_______., and K. R. Gabriel, 1987: Analysis of recording gage data for the Israeli II experiment Part I: Effects of cloud seeding on the components of daily rainfall. J. Appl. Meteor., 26, 913-926. https://doi.org/10.1175/1520-0450(1987)026%3C0913:AORRDF%3E2.0.CO;2
_______, and J. Neumann, 1974a: Rain stimulation and cloud physics in Israel. In Climate and Weather Modification, W. N. Hess, ed., Wiley and Sons, NY, 454-494. No doi.
_______, and _________, 1974b: Modification of subtropical winter cumulus clouds-cloud seeding and cloud physics in Israel. J. Wea. Mod., 6, 203-215. No doi.
_______., and _________, 1976: The second Israeli cloud seeding experiment–the effect of seeding on varying cloud populations. Proc. II WMO Sci. Conf. Weather Modification, Boulder, WMO Geneva, 195-204. No doi.
________, and _________, 1981: The second Israeli randomized cloud seeding experiment: evaluation of results. J. Appl. Meteor., 20, 1301-1311. https://doi.org/10.1175/1520-0450(1981)020%3C1301:TSIRCS%3E2.0.CO;2
_________, and I. Steinhorn, 1974: The role of solid precipitation elements in natural and artificial production of rain in Israel. J. Wea. Mod., 6, 216-228. No doi.
Garstang, M., R. Bruintjes, R. Serafin, H. Orville, B. Boe, W. Cotton, and J. Warburton, 2005: Weather modification. Finding common ground. Bull. Amer. Meteor. Soc., 85, 647-655. https://doi.org/10.1175/BAMS-86-5-647
Givati, A., and D. Rosenfeld, 2005: Separation between cloud-seeding and air-pollution effects. J. Appl. Meteor., 44, 1298-1314. https://doi.org/10.1175/JAM2276.1
________, and D. Rosenfeld, 2009: Comment on “Does air pollution really suppress rain in Israel?”. J. Climate Appl. Meteor., 48, 1733-1750. https://doi.org/10.1175/2009JAMC1902.1
Goldreich, Y., 2003: The Climate of Israel: Observation, Research and Applications. Kluwer Academic/Plenum Publishers, NY. 270pp. No doi.
Grant, L. O., 1968: The role of ice nuclei in the formation of precipitation. Proc. Intern. Conf. Cloud Phys.,Toronto, Amer. Meteor. Soc., 305-310. No doi.
__________, and W. R. Cotton, 1979: Weather modification. Reviews Geophys. Space Phys., 17, No. 7, 1872-1890. No doi.
__________, and R. D. Elliott, 1974: The cloud seeding temperature window. J. Appl. Meteor., 13, 355-363.
Halfon, N., Z. Levin, P. Alpert, 2009: Temporal rainfall fluctuations in Israel and their possible link to urban and air pollution effects. Environ, Res. Lett., 4, 12pp. doi:10.1088/1748-9326/4/2/025001
Hallett, J., and S. C Mossop,1974: Production of secondary ice particles during the riming process. Nature, 249, 26-28. https://doi-org/10.1038/249026a0
Hobbs, P. V., 1969: Ice multiplication in clouds. J. Atmos. Sci., 26, 315-318. https://doi.org/10.1175/1520-0469(1969)026%3C0315:IMIC%3E2.0.CO;2
__________., 2001: Comments on “A critical assessment of glaciogenic seeding of convective clouds to enhance rainfall”. Bull. Amer. Meteor. Soc., 82, 2845-2846. No doi.
Kerr, R. A., 1982: Cloud seeding: one success in 35 years. Science, 217, 519-521. No doi.
Kessler, A., A. Cohen, D. Sharon, 2006: Analysis of the cloud seeding in northern Israel. Areport submitted to the Israel Hydrology Institute and the Israel Water Management of the Ministry of Infrastructure, In Hebrew. 117pp. No doi.
Levi, Y., and D.Rosenfeld, 1996: Ice nuclei, rainwater chemical composition, and static cloud seeding effects in Israel. J.Appl. Meteor., 35, 1494-1501. https://doi.org/10.1175/1520-0450(1996)035<1494:INRCCA>2.0.CO;2
Levin, Z., 1992: The role of large aerosols in the precipitation of the eastern Mediterranean. Paper presented at the Workshop on Cloud Microphysics and Applications to Global Change, Toronto. (Available from Dept. Atmos. Sci., University of Tel Aviv). No doi.
________, 1994: The effects of aerosol composition on the development of rain in the eastern Mediterranean. WMO Workshop on Cloud Microstructure and Applications to Global Change, Toronto, Ontario, Canada. World Meteor. Org., 115-120. No doi.
_________., 2009: On the State of Cloud Seeding for Rain Enhancement. Report to the Energy, Environment and Water Research Center, The Cyprus Institute, Nicosia, Cyprus. 18pp. No doi.
_________., N. Halfon, and P. Alpert: 2011: Reply to the Comment by Ben-Zvi on the paper “Reassessment of rain experiments and operations in Israel including synoptic considerations” Atmos. Res., 99, 593-596. https://doi.org/10.1016/j.atmosres.2010.06.011
_________., E. Ganor, and V. Gladstein, 1996: The effects of desert particles coated with sulfate on rain formation in the eastern Mediterranean. J. Appl. Meteor., 35, 1511-1523. https://doi.org/10.1175/1520-0450(1996)035%3C1511:TEODPC%3E2.0.CO;2
_________., N. Halfon, and P. Alpert, 2010: Reassessment of rain enhancement experiments and operations in Israel including synoptic considerations. Atmos. Res., 97, 513-525. http://dx.doi.org/10.1016/j.atmosres.2010.06.011
_________, S 0. Kirchak, and T. Reisen, 1997: Numerical simulation of dispersal of inert seeding material in Israel using a three-dimensional mesoscale model. J. Appl. Meteor., 36, 474-484.https://doi.org/10.1175/15200450(1997)036%3C0474:NSODOI%3E2.0.CO;2
List, R. L., 1980: The 3rdScientific Conf. on Weather Modification, Clermont-Ferrand, France, Bull. W.M.O., 30, 26-33. No doi.
________, K. R. Gabriel, B. A. Silverman, Z. Levin, and T. Karacostas, 1999: The rain enhancement experiment in Puglia, Italy. Statistical evaluation. J. Appl. Meteor., 38, 281-289. https://doi.org/10.1175/1520-0450(1999)038%3C0281:TREEIP%3E2.0.CO;2
Lutgens, F. K., and E. J. Tarbuck, 1995: The Atmosphere. Prentice-Hall, 462pp. No doi.
Mason, B. J., 1971: The Physics of Clouds. Clarendon Press, Oxford. 671pp. No doi.
__________, 1980: A review of three long-term cloud-seeding experiments. Meteor. Mag., 109, 335-344. No doi.
__________, 1982: Personal Reflections on 35 Years of Cloud Seeding. Contemp. Phys., 23, 311-327. No doi.
Mielke, P. W., Jr., 1979: Comment on field experimentation in weather modification. J. Amer. Statist. Assoc., 74, 87-88. https://doi.org/10.2307/2286729
______________, L. O. Grant, and C. F. Chappell, 1970: Elevation and spatial variation effects of wintertime orographic cloud seeding. J. Appl. Meteor., 9,476-488. Corrigenda,10, 842, 15,801. https://doi.org/10.1175/1520-0450(1970)009%3C0476:EASVEO%3E2.0.CO;2
____________, Grant, L. O., and C. F. Chappell, 1971: An independent replication of the Climax wintertime orographic cloud seeding experiment. J. Appl. Meteor., 10, 1198-1212. https://doi.org/10.1175/1520-0450(1971)010%3C1198:AIROTC%3E2.0.CO;2
_____________., Brier, G. W., Grant, L. O., Mulvey, G. J., and P. N. Rosenweig, 1981: A statistical reanalysis of the replicated Climax I and II wintertime orographic cloud seeding experiments. J. Appl. Meteor., 20, 643-659. https://doi.org/10.1175/1520-0450(1981)020%3C0643:ASROTR%3E2.0.CO;2
Moran, J. M., M. D. Morgan, and P. M. Pauley, 1991: The Essentials of Weather,Prentice-Hall, 351pp. No doi available.
Mossop, S. C., 1978: Some factors governing ice particle multiplication in cumulus clouds. J. Atmos. Sci., 35, 2033–2037. https://doi.org/10.1175/1520-0469(1978)035%3C2033:SFGIPM%3E2.0.CO;2
_____________, 1985: The origin and concentration of ice crystals in clouds. Bull. Amer. Meteor. Soc., 66, 264-273. https://doi.org/10.1175/1520-0477(1985)066%3C0264:TOACOI%3E2.0.CO;2
National Academy of Sciences-National Research Council, 1973: Weather and Climate Modification: Progress and Problems, T. F. Malone, Ed., Government Printing Office, Washington, D. C., 258 pp. No doi.
________________________________________________, 2003: Critical Issues in Weather Modification Research. Committee on the Status of and Future Directions in U.S. Weather Modification Research and Operations, M. Garstang, Chairman. 123pp. No doi.
Neiburger, M., J. G. Edinger, W. D. Bonner, 1982: Understanding Our Atmospheric Environment. W. H. Freeman and Company. No doi.
Neumann, J., and E. Shimbursky, 1972: On the distribution of a ratio of interest in single-area cloud seeding experiments. J. Appl. Meteor., 11, 370-375. https://doi.org/10.1175/1520-0450(1972)011%3C0370:OTDOAR%3E2.0.CO;2
___________, K. R. Gabriel, and A. Gagin, 1967: Cloud seeding and cloud physics in Israel: results and problems. Proc. Intern. Conf. on Water for Peace. Water for Peace, Vol. 2, 375-388. No doi.
Nirel, R., and D. Rosenfeld, 1994: The third Israeli rain enhancement experiment-An intermediate analysis. Proc. Sixth WMO Scientific Conf. on Weather Modification, Paestum, Italy, World Meteor. Org., 569-572. No doi.
__________ and _________, 1995: Estimation of the effect of operational seeding on rain amounts in Israel. J. Appl. Meteor., 34, 2220-2229. https://doi.org/10.1175/1520-0450(1995)034%3C2220:EOTEOO%3E2.0.CO;2
Patrich, J., and A. Gagin, 1970: Ice crystals in cumulus clouds—preliminary results. Preprints, Int. Conf. Meteor., Tel Aviv. No doi available.
Ramanathan, V., P. J. Crutzen, J. T. Kiehl, and D. Rosenfeld, 2001: Aerosols, climate and the hydrological cycle. Science, 294, 2119-2124. https://doi-org/10.1126/science.1064034
Rangno, A. L., 1988: Rain from clouds with tops warmer than -10 C in Israel. Quart J. Roy. Meteor. Soc., 114, 495-513. https://doi-org/10.1002/qj.49711448011
__________, 2000: Comment on “A review of cloud seeding experiments to enhance precipitation and some new prospects.” Bull. Amer. Meteor. Soc., 81, 583-585. No doi
_________, and Hobbs, P. V., 1988: Criteria for the development of significant concentrations of ice particles in cumulus clouds. Atmos. Res., 22, 1-13. No doi.
__________, and ___________, 1993: Further analyses of the Climax cloud-seeding experiments. J. Appl. Meteor., 32, 1837-1847. https://doi.org/10.1175/1520-0450(1993)032%3C1837:FAOTCC%3E2.0.CO;2
___________, and __________, 1995a: A new look at the Israeli cloud seeding experiments. J. Appl. Meteor., 34, 1169-1193. https://doi.org/10.1175/1520-0450(1995)034%3C1169:ANLATI%3E2.0.CO;2
___________, and _________, 1995b: Reply to Gabriel and Mielke. J. Appl. Meteor., 34, 1233-1238. https://doi.org/10.1175/1520-0450(1995)034%3C1233:R%3E2.0.CO;2
___________., and ___________, 1997a: Reply to Rosenfeld. J. Appl. Meteor., 36, 272-276. https://doi.org/10.1175/1520-0450(1997)036%3C0272:R%3E2.0.CO;2
___________., and ___________, 1997b: ComprehensiveReply to Rosenfeld, Cloud and Aerosol Research Group, Department of Atmospheric Sciences, University of Washington, 25pp. (http://carg.atmos.washington.edu/sys/research/archive/1997_comments_seeding.pdf)
___________, and ___________, 1997c: Reply to Ben-Zvi. J. Appl. Meteor., 36, 257-259. https://doi.org/10.1175/1520-0450(1997)036%3C0257:R%3E2.0.CO;2
___________, and ___________, 1997d: Reply to Dennis and Orville. J. Appl. Meteor., 36, 279. https://doi.org/10.1175/1520-0450(1997)036%3C0279:R%3E2.0.CO;2
___________., and ___________, 1997e: Reply to Woodley. J. Appl. Meteor., 36, 253. https://doi.org/10.1175/1520-0450(1997)036%3C0253:R%3E2.0.CO;2
Rosenfeld, D., 1980: Characteristics of rain cloud systems in Israel as derived from radar data and satellite images. Ms. Thesis, Hebrew University of Jerusalem (in Hebrew) 129pp.
__________, 1997: Comment on “Reanalysis of the Israeli Cloud Seeding Experiments”, J. Appl. Meteor., 36, 260-271.https://doi.org/10.1175/1520-0450(1997)036%3C0260:COANLA%3E2.0.CO;2
___________, 1998: The third Israeli randomized cloud seeding experiment in the south: evaluation of the results and review of all three experiments. Preprints, 14th Conf. on Planned and Inadvertent Wea. Modif., Everett, Amer. Meteor. Soc. 565-568. No doi.
___________., and H. Farbstein, 1992: Possible influence of desert dust on seedability of clouds in Israel. J. Appl. Meteor., 31, 722-731. https://doi.org/10.1175/1520-0450(1992)031%3C0722:PIODDO%3E2.0.CO;2
___________ and A. Gagin, 1989: Factors governing the total rainfall yield from continental convective clouds. J. Appl. Meteor., 28, 1015-1030.https://doi.org/10.1175/1520-0450(1989)028%3C1015:FGTTRY%3E2.0.CO;2
____________, and R. Nirel, 1996: Seeding effectiveness—the interaction of desert dust and the southern margins of rain cloud systems in Israel. J. Appl. Meteor., 35, 1502-1510. https://doi.org/10.1175/1520-0450(1996)035%3C1502:SEIODD%3E2.0.CO;2
Sax, R. I., S. A. Changnon, L. O. Grant, W. F. Hitchfield, P. V. Hobbs, A. M. Kahan, and J. Simpson, 1975: Weather modification: Where are we now and where are we going? An editorial overview. J. Appl. Meteor., 14, 652-672. https://doi.org/10.1175/1520-0450(1975)014%3C0652:WMWAWN%3E2.0.CO;2
Sharon, D., 1978: Rainfall fields in Israel and Jordan and the effect of cloud seeding on them. J. Appl. Meteor., 17, 40-48. https://doi.org/10.1175/1520-0450(1978)017%3C0040:RFIIAJ%3E2.0.CO;2
_________, 1990: Meta-analytic reappraisal of statistical results in the environmental sciences: the case of a hydrological effect of cloud seeding. J. Appl. Meteor., 29, 390-395. https://doi.org/10.1175/1520-0450(1990)029%3C0390:MAROSR%3E2.0.CO;2
_________, A. Kessler, A. Cohen, and E. Doveh, 2008: The history and recent revision of Israel’s cloud seeding program. Isr. J. Earth Sci., 57, 65-69. https://DOI.org/10.1560/IJES.57.1.65.
Silverman, B. A., 1986: Static mode seeding of summer cumuli–a review. In Precipitation Enhancement–A Scientific Challenge, Meteor. Monog., 21, No. 43, 7-20. https://doi.org/10.1175/0065-9401-21.43.7
_____________, 2001. A critical assessment of glaciogenic seeding of convective clouds for rainfall enhancement. Bull. Amer. Meteor. Soc.,82, 903-924. https://doi.org/10.1175/1520-0477(2001)082%3C0903:ACAOGS%3E2.3.CO;2
Simpson, J. S., 1979: Comment on “Field experimentation in weather modification.” J. Amer. Statist. Assoc., 74, 95-97. https://doi.org/10.2307/2286732
Tukey, J. W., Jones, L. V., and D. R. Brillinger, 1978a: The Management of Weather Resources, Vol. I, Proposals for a National Policy and Program. Report of the Statistical Task Force to the Weather Modification Advisory Board, Government Printing Office. 118pp. No doi.
__________., D. R. Brillinger, and L. V. Jones, 1978b: Report of the Statistical Task Force to the Weather Modification Advisory Board,Vol. II. U. S. Government Printing Office, pE-3. No doi.
Vali, G., L. R. Koenig, and T. C. Yoksas, 1988: Estimate of Precipitation Enhancement Potential for the Duero Basin of Spain. J. Appl. Meteor., 27, 829-850. https://doi.org/10.1175/1520-0450(1988)027%3C0829:EOPEPF%3E2.0.CO;2
Wallace, J. M., and P. V. Hobbs, 1977: Atmospheric Science: An Introductory Survey. Academic Press, 467 pp. No doi.
Woodley, W., 1997: Comments on “A new look at the Israeli Randomized cloud seeding experiments.” J. Appl. Meteor., 36, 250-252. https://doi.org/10.1175/1520-0450(1997)036%3C0250:COANLA%3E2.0.CO;2
World Meteorological Organization, 1986: Statement on weather modification. Bull. W. M. O.,35, 45-46. No doi.
___________________________, 1988: Statement on weather modification. Bull. W. M. O., 37, 140-144. No doi.
___________________________, 1992: Statement on planned and inadvertent weather modification. WMO, Geneva. Approved July 1992. No doi.
Wurtele, Z. S., 1971: Analysis of the Israeli cloud seeding experiment by means of concomitant meteorological variables. J. Appl. Meteor., 10, 1185-1192. https://doi.org/10.1175/1520-0450(1971)010%3C1185:AOTICS%3E2.0.CO;2
Young, K. C., 1993: Microphysical Processes in Clouds. Oxford University Press, 200 Madison Avenue, New York, New York 10016, 427pp. No doi.
———————————————————————————Endnotes
1Ben-Zvi and Fanar (1996) contradicted the results of Gagin and Gabriel (1987) reporting that rainfall intensities had been increased on seeded days in Israel-2, contrary to expectations due to kind of seeding (termed, “static”) that had been conducted.
[2]Due to the likely contribution of probe shattering artifacts in the Levin measurements, values half those reported by Levin are also plotted in Figure 3, an overestimate of artifact contributions.
[3]Updated in RH95a, Figure 12.
[4]The HUJ experimenters have conducted many flights into Israeli clouds since 1990 to determine their microstructural nature and cloud seeding potential but, in spite of their critical importance for cloud seeding, have not been able to report ice particle concentrations or the rapidity at which they develop due to carrying imaging probes on their aircraft that are not capable of measuring them accurately (Freud et al. 2015; D. Rosenfeld, 2019, private communication in his review of this manuscript).
[5]The HUJ experimenters changed evaluation techniques from Israel-1 to Israel-2 in reporting a second seeding success and did not pursue the several methods of evaluation outlined in GN74 and here.
[6]“Aftersome 2 ½ seasons of operational seeding (i. e.,“randomized”—author’s insertion for clarity)experience, it was noticed that flying was effectively limited in such a way as to affect only the interior parts of the two areas.” This was repeated by Gabriel (1979).
[7]The random draw sequence for Israel-2 was markedly different from than the one for Israel-1. In Israel-2 long strings of the same random decision occurred whereas in Israel-1 they did not.
[8]In 1969 the Israel Rain Committee, formed by Mekorot, Israel’s national water company, was responsible for overseeing the design of the Israel-2 experiment. They recommended that rainfall data from Lebanon be incorporated in the evaluations of Israel-2 when it was completed. This did not take place in the experimenters’ many published evaluations; such data may not have been available to them.
[9]Goldreich (2003) reported that operational seeding took place during the 1968-69 rain season that fell between Israel-1 and 2.
[10]The lack of timely reporting of indications of decreased rain on seeded days in Israel-3 made Gabriel’s (1967a) statement appear as “prophesy” for his own experiments; that negative seeding results may not be reported at all.
[11]In 1988 the AMS dropped its “Code of Ethics” that described the requirements and attributes of professional conduct as a member, downgrading those elements to, “guidelines”, a word synonymous with “suggestions.”
[12]G. Vali, 1986; Mason, Sir B. J., 1997, personal communications, available on request or go here: https://cloud-maven.com/my-life-in-cloud-seeding-1970-2020/